Shigeru Horii
Professor, Doctor of Engineering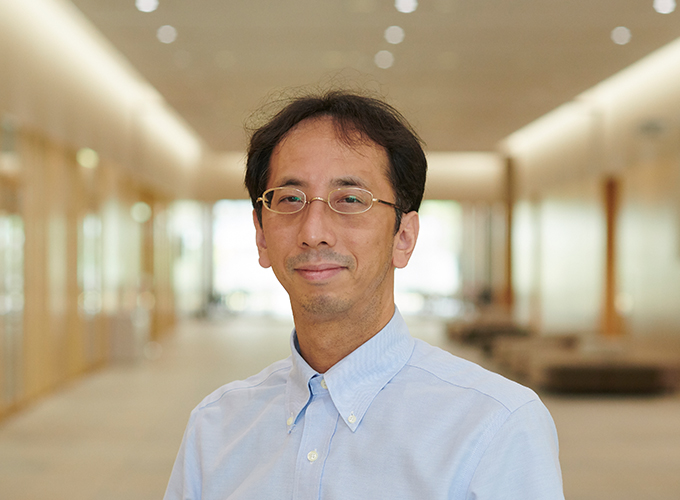
- horii.shigeru
- Areas of Research
- Materials Science, Solid-state Physics, Ceramics, Strong Magnetic Field Science, Superconductivity
- Profile
- Research
-
Dr. Shigeru Horii obtained his B.E., M.E. and Dr. Eng. from Nagoya University in 1994, 1996 and 1999, respectively. Previously, he worked at the University of Tokyo from 1999 to 2009, the Kochi University of Technology from 2009 to 2013, and Kyoto University from 2013 to 2019. An experienced scholar, he has worked for more than 25 years in the academic field of functional oxide materials with low-dimensional crystal structure on the basis of materials science and solid state physics and has published more than 300 papers related to those fields. Furthermore, he has extensive experience as a committee member of domestic scientific societies and international conferences.
Dr. Horii is currently engaged in the research of magnetic alignment in layered functional materials. In particular, he focuses on the development of linear-drive type of modulated rotating magnetic fields and precise understanding of magnetic alignment behavior in dispersed mediums. He is also interested in research related to magnetic field control using a simulation technique for the magnetic alignment and materials science of superconductivity and feeble magnetic materials.
As a professor of KUAS Faculty of Engineering, Dr. Horii is involved with basic subjects in physics. He is excited about the big challenge of contributing to the fostering the next generation of engineers taking part in KUAS’ unique engineering program. Dr. Horii welcomes all students interested in the fields of electronics, mechanics and related sciences.
-
Materials Innovation through Magnetic Flux Control
There is a common misconception that some materials and substances are “non-magnetic” and do not respond to magnets at all, but in truth, everything is magnetic to a certain extent.
In primary school, most of us learn that certain objects like coins or water do not show responses to magnets, and their non-magnetism is presented as a fact in children’s textbooks. However, when introduced to magnetic fields that are 100 or 1000 times stronger, these substances do, in fact, demonstrate a magnetic response.
When exposed to a sufficiently strong magnetic field, water is shown to be magnetically repulsive and aluminum to be attractive. The magnets we use in everyday life are not nearly strong enough to produce a visually observable reaction, but even so, the response of things like water and aluminum to magnets should be understood as “extremely weak” rather than “unresponsive”. Moreover, one can recognize that all materials and substances show some magnetic properties, and these should be referred to as “feeble magnetic substances” rather than “non-magnetic substances”.
Given that all materials are magnetic on some level, by introducing them to a magnetic field of sufficient intensity, we can expect them to demonstrate some visually observable response. In 1994, a Japanese company developed cryogen-free superconducting solenoidal electromagnets capable of producing a high magnetic field of 10 teslas while only requiring electric refrigeration, thereby foregoing the need for rare and expensive liquid helium. Thus, experiments using high-intensity magnetic fields were made much easier to perform, and allowed more researchers to contribute to the creation of a new field: “magnetoscience”.
At the current stage, the magnetoscience research group at KUAS focuses on material-production processes, with magnetic alignment being one of the main research topics. Dr. Horii’s laboratory has a sophisticated superconducting electromagnet, which can generate a 10 tesla-class magnetic field and a newly developed arranged magnet specialized for magnetic alignment work. His lab also studies the crystal orientation of functional ceramics with anisotropic crystal structures. Dr. Horii and collaborators group also focus on the development of materials appropriate for magnetic alignment. Enhancing the magnetic anisotropies of substances directly leads to a reduction in the magnetic field required for magneticalignment, which is strongly related to the specification and cost of magnets. In this context, knowledge of solid-state chemistry and inorganic chemistry is useful.
The photograph below is an example of a tri-axial magnetic alignment using a modulated rotating magnetic field (MRF) generated by a superconducting electromagnet. The photograph shows a magnetically aligned sample of oxide-based superconductor crystals cured in transparent epoxy resin under a 10-tesla MRF at room temperature. The shape of the crystals is a plate-like cuboid and, in the photograph, the surfaces of the crystals with the largest area are aligning along the longest axis. All of the crystals are well aligned, and this is obvious evidence of tri-axial magnetic alignment under MRF. In the case of epitaxy-based crystal growth, precise control of temperature and highly aligned templates are indispensable to the obtainment of such crystals. However, magnetic alignment can be achieved at room temperature without a highly aligned template or precise temperature control. This magnetoscientific technique, based on superconducting electromagnets, has the potential to become a standard production process for materials and to contribute to the next generation of materials innovation.